Research Projects - Copper Roof Stormwater Runoff
The State of Connecticut Department of Environmental Protection (DEP) had found that most of its major rivers exceed the copper water quality criteria several times a year, leading to concerns about the potential adverse effect of copper on aquatic life. The DEP was considering limiting the use of copper in architectural applications. This resulted in the CDA and the DEP working together to develop a better understanding of the mobility, fate and forms of copper in an urban watershed and the relationship between copper exceeding regulatory limits, and the lack of, or degree of toxicity observed in freshwater systems.
The copper content and flow of stormwater runoff was measured at five locations within the 46.5 hectare watershed at the University of Connecticut (UCONN) campus at Storrs, which included a UNS Alloy C11000 1800 m 2 copper roof installed in 1992 6,7 , which is brown in color as a result of exposure to the atmosphere over the time period since it was installed. Copper concentration in stormwater was also measured on a 1258m 2 copper roof installed in 1931 at Trinity College, in nearby Hartford, Connecticut, which, however, resides in another watershed. The latter roof has developed a fully mature green patina.
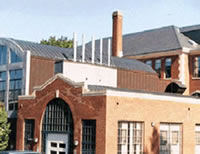
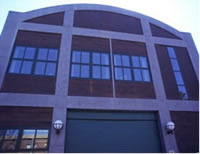
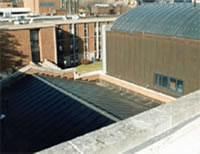
The 1800m 2 copper roof at UCONN was installed in 1992 on the Castleman Engineering Building. It consists of a segment of an arch, as can be seen in Figures 9a, b and c. The overview of the watershed and its five sampling stations is shown in Figure 10. The mean and standard deviation, for total, dissolved and ionic copper concentration, as measured over 16 storms at Sampling Stations 1 through 6 are listed in Table 10. Sampling Station 6 is located at the old roof at Trinity College. The average rainfall was 20.1 mm during the 16 storm events, which lasted an average of 7.9 hours. Dissolved copper is defined as a measurement of all the copper passing through a 0.45 mm filter and includes both fine (<0.45µm) particles and ionic copper. Thus dissolved copper is always greater than and should not be considered to be the same as ionic copper when fine (<0.45 µm) copper particulates are present. Sampling Station 6 and its associated data, will be discussed separately because this 1258m 2 roof, which was installed in 1931, resides in a different watershed, on the annex to the chapel at Trinity College in Hartford, Connecticut.
A comparison of the copper concentrations observed at the New Roof (Sampling Station 1) and the Roof Drain (Sampling Station 2), show that both total and dissolved copper concentration are reduced by about 63% while ionic copper concentration is reduced by 54%, as listed in Table 10. Since the non-copper area of the roof is 18% of the total area, this reduction can only account for, at most, approximately 20% or a fifth of the observed reduction. It is hypothesized that the balance, or approximately 80% of the observed reduction, is attributable to interaction with the cast iron and concrete drain pipes. Dissolved organic carbon (DOC) in the stormwater, as well as other organic matter also plays a role in reducing copper concentrations. This interaction of copper with the drainage system has important implications in regard to mitigation of copper runoff. Concrete has been shown to collect copper from roof stormwater runoff 8 . Others have reported that iron surfaces have been shown to be responsible for a significant reduction of copper levels over a short period of time 9,10 . Decaying leaves and other organic matter may also complex with ionic copper. As an aside, a commercial product, an organic granular stormwater filter media for metals based upon humus from collected deciduous leaves is available 11 . Thus passing copper roof stormwater runoff through filters containing iron filings, or decaying organic matter, may be an effective way to reduce copper release to the environment.
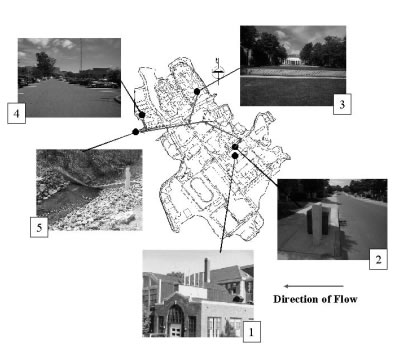
Sampling Station 3, the Lawn Area and Sampling Station 4, the Parking Lot exhibit total and dissolved copper concentrations values which are approximately two orders of magnitude lower than that of Sampling Station 1, the New Copper Roof, and Sampling Station 2, the Roof Drain, as listed in Table 10. Potential sources of copper at Sample Station 3, the Lawn Area include the rain itself as well as pesticides and synthetic fertilizers. Possible sources of copper at Sample Station 4, the Parking Lot, are rain, automobile fluids and brake pad dust.
Sampling Station | Total Copper(µg/L) | Dissolved Copper(µg/L) | IonicCopper(µg/L) |
---|---|---|---|
1. New Roof | 3633 +/- 1760 | 3338 +/- 1520 | 1733 +/- 900 |
2. Roof Drain | 1340 +/- 820 | 1210 +/- 840 | 802 +/- 170 |
3. Lawn Area | 20 +/- 8 | 9 +/- 2 | <0.05 |
4. Parking Lot | 16 +/- 6 | 8 +/- 2 | 0.39 +/- 0.2 |
5. Stream | 46 +/- 26 | 14 +/- 7 | <0.05 |
6. Old Roof | 1461 +/- 840 | 1338 +/- 760 | 620 +/- 380 |
As the stormwater flows on through the system and over the weir at the stream exiting the UCONN Campus, an even more dramatic reduction in ionic copper is seen. When the ionic copper concentrations of Sampling Stations 1 The New Copper Roof, and 2 the Roof Drain are compared with Sampling Station 5 the Stream, the reduction is almost 100%, can also be seen in Table 10. This is a result of not only dilution but also the strong tendency of ionic copper to complex and bind. The ionic copper is the form of copper that adversely affects sensitive aquatic organisms. Binding or complexing renders the ionic copper unavailable and thus benign to the environment. Note the 0.39 µg/L dissolved copper concentration for Sampling Station 4, as listed in Table 10. This is attributed to inadequate or incomplete complexing of ionic copper (which is a component of dissolved copper), in the Parking Lot.
Some of the key parameters measured in the collected rain, and at the five sampling stations within the watershed, are shown in Table 11. The mean pH of the rain is 4.7, but increases to 6.2 at Sampling Station 1, the New Copper Roof Downspout, and increases further to 7.2 at Sampling Station 5, the Stream. When acid rain falls on the copper roof, it releases ionic copper. It has been reported12 that the first rain to fall has the lowest pH and pH subsequently increases. This low initial pH of the acid rain also contributes to the high initial reading, or "first flush" observed in Table 12, as does the dissolution of neutral copper salts formed by acid gases in the air.
Sampling Station | Initial (µg/L) | Mean (µg/L) |
---|---|---|
1. New Roof | 6703 +/- 3801 | 3633 +/- 1760 |
2. Roof Drain | 2296 +/- 1367 | 1340 +/- 820 |
6. Old Roof | 3838 +/- 1561 | 1461 +/- 840 |
DOC, which was present at all five sampling stations ( Table 11), reduces ionic copper in solution by complexing it. Furthermore, both calcium and magnesium compete with ionic copper for binding sites on the gills of fish or other biotic ligands 13,14 , which are binding sites at which ionic copper can potentially cause toxicity. Hence, high pH values, as well as high hardness and DOC all favor binding and/or complexing ionic copper resulting in the reduction in the availability and bioavailability of ionic copper. This was confirmed in the acute toxicology tests performed on neonatal Daphnia pulex, a small aquatic organism about the size of a head pin, which is very sensitive to ionic copper, and is shown in Figure 11. Acute toxicity values are commonly expressed in terms of LC 50, which is the concentration of ionic copper in this case, at which 50% survival is seen after a 48 hour exposure. By convention, 50% survival (after 48 hours) equates to an LC 50 of 100%.
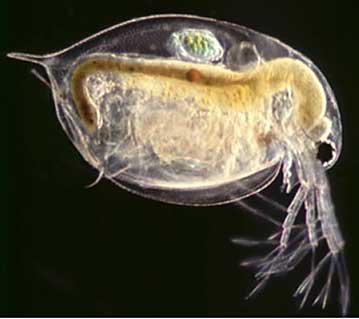
At Sampling Stations 1 and 2, which drain the copper roof and where ionic copper concentration is the highest, survival, as expected, was very low, and the LC 50 is < 0.62% at both locations, as shown at the bottom of Table 11. At Sampling Stations 3, 4 and 5 which are, respectively, the Lawn Area, the Parking Lot, and the Stream, ionic copper concentrations are below 1 µg/L and LC 50 > 100% as is also listed in Table 11, and most samples exhibited 100% survival. The ionic copper coming off the roof does not want to remain in the ionic state and readily binds to the cast iron and concrete piping system. DOC, which is found at all sampling stations, also complexes with the ionic copper. As the water flows through the storm system, the pH and hardness increase. Furthermore, both magnesium and calcium compete with the ionic copper at biotic ligands. The net result of these interactions is that the ionic copper is either reduced or rendered benign to the environment. Thus the bioavailability of the copper is reduced, or less copper in the potentially environmentally harmful is available.
The Old Copper Roof, which was installed in 1931 at the Chapel Annex at Trinity College in Hartford, Connecticut, is shown in Figure 12(a). Sampling Station 6, the Old Copper Roof Downspout is shown in Figure 12(b). In Table 10, it is apparent that total copper, dissolved copper and ionic copper concentrations for the old roof are less than half of the corresponding values for the new roof.
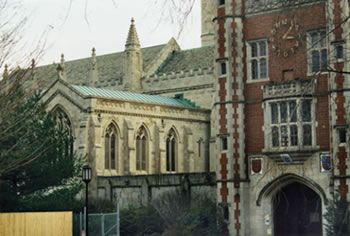
Figure 12(a). Old copper roof installed in 1931 on Chapel Annex at Trinity College.
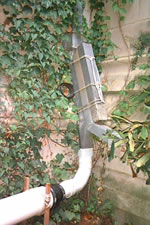
Figure 12(b)Old copper roof downspout and PVC sampling port.
Linear striations which may be attributable to mill processing and fabrication are still visible on the new roof, as can be seen in the Scanning Electron Microscope (SEM) image shown in Figure 13(a). In contrast, the SEM image for the old roof shown in Figure 13(b) shows a uniformly mottled appearance. X-Ray diffraction (XRD) confirms that cuprite (Cu 2O) is present on the new roof, which is brown in color. The old roof contains both cuprite and Brochantite, (Cu4SO4 (OH)6), and small amounts of Posnjakite, (Cu 4SO 4 (OH) 6H 2O), and has a fully mature green patina. This is consistent with the observations of others15 and as expected, forms above the cuprite. Kramer reported 12 that the corrosion product thought to be the terminal phase formed on the copper has lower solubility than its precursor phases. Thus Brochantite, the terminal phase 15 , should exhibit somewhat lower solubility and, therefore contribute less to runoff than cuprite. This favors and is consistent with the observation that copper concentrate in runoff is lower on the old roof when compared to the new roof, as listed in Table 10. Others have also attributed a reduction in copper runoff to patina formation 16,17 .
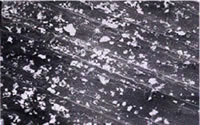
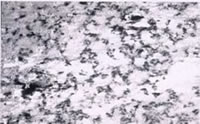
Conclusions from the Copper Stormwater Runoff Research Project
The major conclusions are:- ionic copper is the form of which has the potential to be toxic to aquatic organisms, but this is managed so it is no longer harmful.
- ionic copper is readily transformed by binding and complexing.
- ionic copper is sufficiently concentrated so as to be acutely toxic, to Daphnia pulex, at the roof downspout and drain at the street, but toxicity is not observed in the balance of the watershed.
- simply allowing the stormwater to flow through the drainage system lowers its ionic copper concentration to the level where environmental harm is no longer observed.
- ionic copper readily binds to cast iron and concrete pipe and interacts with DOC, and organic debris.
- filters made of iron filings or organic matter should readily transform and reduce ionic copper.
- copper in stormwater is no longer toxic by the time it reaches the stream.
- the old copper roof releases less than half the amount of copper in comparison to the new copper roof.
CONCLUSIONS
In conclusion, I trust this paper has demonstrated that the copper industry is involved in a variety of activities, which can be useful in solving corrosion problems.
ACKNOWLEDGEMENT
Thanks is given to the Copper Development Association and the International Copper Association and their member companies, for without their support, the information presented in this paper would not as so readily available.
REFERECES
-
B. Bouanger and N.P. Nikolaidis, Journal of Am. Water Res. Ass., (In Review) 2001.
-
H. T. Michels, B. Bouanger and N.P. Nikolaidis, Copper Roof Stormwater Runoff- Corrosion and the Environment, Paper No. 02225, CORROSION 2002, NACE International, Houston, TX.
-
R. Sundberg, The Fate of Copper Release from the Vasci Ship Museum Metall, 52. Jahrgang. Nr. 4/98.
-
T. Shokes and G. Moller, Environ. Sci. Technical 1999.
-
K. Cantrell, D. Kaplan and T. Wietsman, Environ. Sci. Technol., 42: 201-212 19959.
-
Stormwater Management Inc., CSF® Leaf Media, Portland, Oregon 2001.
-
S.D. Kramer, S.A. Matthes, B.S. Covino, Jr., S.J. Bullard and G.R. Holcomb, ASTM Environmental Factor Affecting Atmosphere Corrosion of Copper, ASTM Symposium, Outdoor and Indoor Corrosion, Phoenix, AZ, 2001.
-
L. Hollis, L. Muench and R.C. Playle, J. Fish Bio.,50, 703-720, 1997.
-
J. S. Meyer, R. C. Santore, J. P. Babbit, L. D. Debrey, C. J. Boese, P. R. Paquin, H. E. Allen, H. L. Bergman and D. M. DiToro Environ. Sci. Technol., 33, 913-916, 1999.
-
1C. Leygraf and T. Graedel, Atmospheric Corrosion, p.141 (Electrochemical Society Series), John Wiley, New York, 2000.
-
T. E. Graedel, K. Nassau and J. P. Franey, Copper Patinas in the Atmosphere -1, Introduction, Corrosion Sci., 27 (7), 639-657, 1987.
-
S. Oescg and P. Hemgartner, Mat. Corros., 47: 425, 1996.
Previous... |